Heart Rate Variability
- Graham Exelby
- Apr 11, 2023
- 9 min read
Figure 1: Heart Rate variability
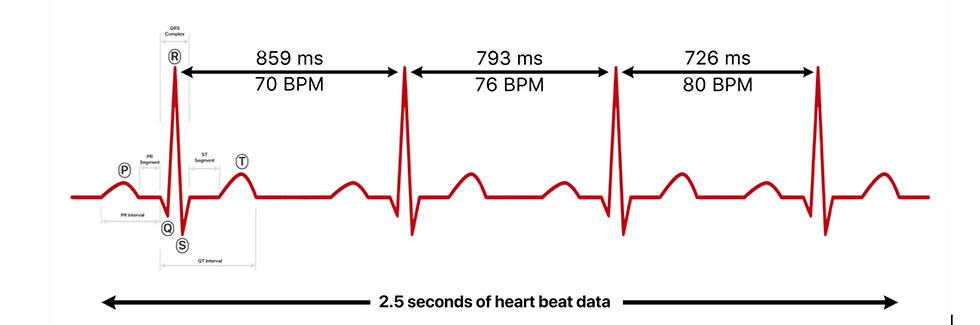
Figure 2: HRV by gender and age
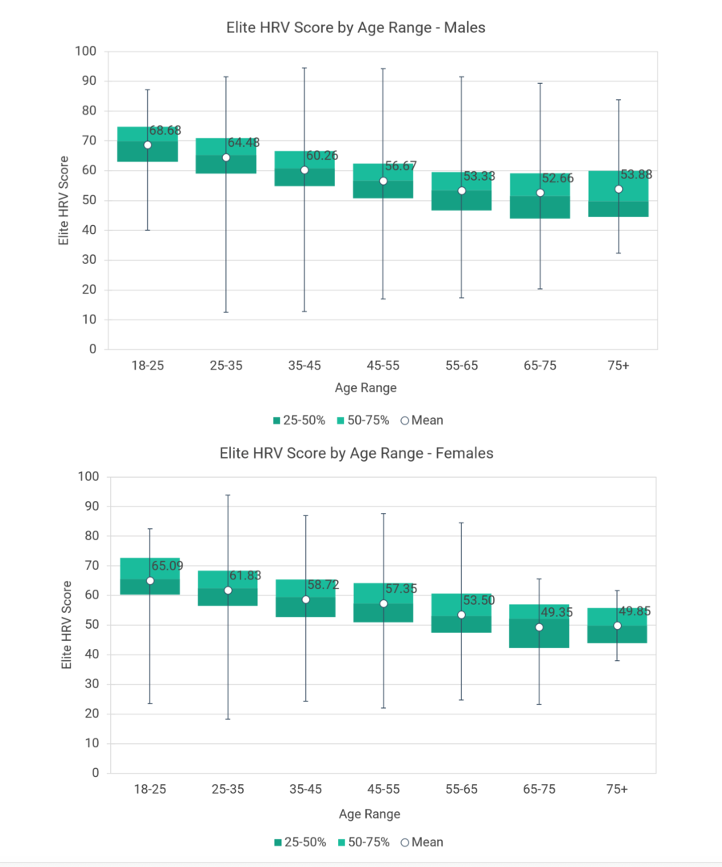
Source: Jason Moore: Normative HRV Scores by Age and Gender [Heart Rate Variability Chart] https://elitehrv.com/normal-heart-rate-variability-age-gender (2)
SDNN by Age
Figure 3: SDNN by age
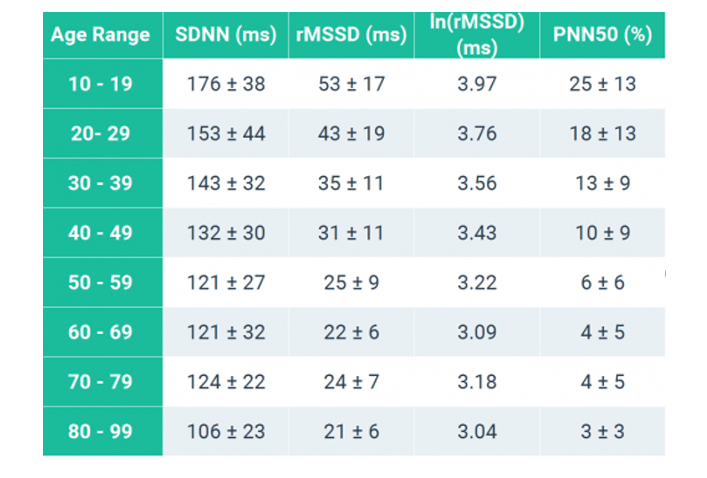
Source: Umetani K, Singer DH, McCraty R, Atkinson M. Twenty-four hour time domain heart rate variability and heart rate: relations to age and gender over nine decades. J Am Coll Cardiol. 1998 Mar 1;31(3):593-601. doi: 10.1016/s0735-1097(97)00554-8. PMID: 9502641.(3)
Jason Moore from Elite describes: “Heart Rate Variability is a measure which indicates how much variation there is in your heartbeats within a specific timeframe. The unit of measurement is milliseconds (ms). If the intervals between your heartbeats are rather constant, your HRV is low. If their length variates, your HRV is high.
There are different ways to calculate HRV, but they all have to do with the amount of variation in the intervals between heartbeats.
R-R INTERVALS AND INTERBEAT INTERVALS
How you calculate heart rate variability depends on what technology you use. Using ECG, or electrocardiogram, it’s typically the R peak in the QRS complex that marks a heartbeat. Hence, the intervals between heartbeats are called R-R intervals.
The autonomic nervous system regulates very important systems in our body, including heart and respiration rate and digestion. The autonomic nervous system has a parasympathetic (rest) and a sympathetic (activation) branch. Heart rate variability is an indicator that both branches are functioning – the parasympathetic in particular.
THE BASICS
To understand HRV we first need to understand our nervous system and heart rate. Heart rate variability can be traced back to our autonomic nervous system.
Intrinsic heart rate is measured in the condition in which neither parasympathetic nor sympathetic regulation is present. When completely blocked from autonomic regulation, a healthy heart contracts at a rate of about 100 beats per minute (the number is individual, though).
Parasympathetic regulation lowers your heart rate from the intrinsic level, giving more room for variability between successive heartbeats.
Parasympathetic regulation causes almost immediate changes that affect only a few beats at a time, after which heart rate returns towards the intrinsic rate.
Sympathetic regulation elevates your heart rate from the intrinsic level, and there is less room for variability between successive heartbeats. Sympathetic regulation affects several consecutive heart beats.
Put these together and we can formulate a rule that when the rest-related parasympathetic branch is active and the sympathetic branch is inactive, your heart rate is lower and HRV higher.
Put these together and we can formulate a rule that when the rest-related parasympathetic branch is active and the sympathetic branch is inactive, your heart rate is lower and HRV higher. Factors such as stress can lead to the withdrawal of parasympathetic activity, or activation of sympathetic branch even when you are resting, both leading to elevated heart rate and lowered HRV.”(2)
Figure 4: The prefrontal cortex regulation and inhibition of activity in limbic structures which act to suppress parasympathetic activity and activate sympathetic circuits.

Source: Stevan Nikolin, Tjeerd W. Boonstra, Colleen K. Loo, Donel Martin - Nikolin S, Boonstra TW, Loo CK, Martin D (2017) Combined effect of prefrontal transcranial direct current stimulation and a working memory task on heart rate variability. PLoS ONE 12(8): e0181833. https://doi.org/10.1371/journal.pone.0181833, CC BY 2.5, https://commons.wikimedia.org/w/index.php?curid=61549870
Figure 5: Brain regions relevant to the neurovisceral integration model

Figure 5 : Brain regions relevant to the neurovisceral integration model. PC, prefrontal cortex; CC, cingulate cortex; Hyp, hypothalamus; Ins, insula; Amy, amygdala; BS, brainstem.
Source: Stevan Nikolin, Tjeerd W. Boonstra, Colleen K. Loo, Donel Martin - Nikolin S, Boonstra TW, Loo CK, Martin D (2017) Combined effect of prefrontal transcranial direct current stimulation and a working memory task on heart rate variability (1)
Steven Nikolin et al describe: “Prefrontal cortex activity has been associated with changes to heart rate variability (HRV) via mediation of the cortico-subcortical pathways that regulate the parasympathetic and sympathetic branches of the autonomic nervous system. Changes in HRV due to altered prefrontal cortex functioning suggests that prefrontal hyperactivity increases parasympathetic tone and decreases contributions from the sympathetic nervous system. (1)
Heart rate variability (HRV), an index of cardiac adaptation to allostatic load, is known to be regulated by the prefrontal cortex such that changes in prefrontal cortex functioning show measurable effects on HRV. Alteration of prefrontal cortex activity has been independently demonstrated to modulate HRV using both non-invasive brain stimulation, and cognitive tasks reliant on prefrontal functioning . However, several outstanding questions remain. Specifically, whether brain stimulation of the prefrontal cortex alters HRV at rest, whether these effects outlast the period of stimulation, and what the combined effect of both prefrontal cortex brain stimulation and a task known to predominantly engage regions of the prefrontal cortex is on the autonomic nervous system. The prefrontal cortex is known to modulate brain regions involved in the regulation of autonomic nervous system activity, such as heart rate.(1)
Both parasympathetic and sympathetic branches of the nervous system are mediated by cortical-subcortical pathways which involve the prefrontal cortex, the anterior cingulate cortex, the insula, the hypothalamus, and the brainstem. The prefrontal cortex regulates and tonically inhibits activity in limbic structures which act to suppress parasympathetic activity and activate sympathetic circuits–see Figure above.
Variation in the output of these two branches of the autonomic system produces heart rate variability (HRV), a measure of autonomic nervous functioning . Therefore, activation of the prefrontal cortex results in change to HRV, which can be thought of as a measure of the aggregate effect of activity in a complex brain network, regulated top-down by the prefrontal cortex. According to the model, hyper-activation of the prefrontal cortex inhibits the sympathoexcitatory circuit of the amygdala, which is known to have outputs relevant to autonomic regulation. This in turn reduces sympathetic activity and parasympathetic suppression, culminating in a reduction in heart rate.
Nikolin et al concluded that “Measures of HRV present as potential indices for activation of cortico-subcortical neural circuitry following activation of the prefrontal cortex both during vagally dominated periods of resting-state activity and sympatho-adrenal mediated task-related activity. This network is shared for both cognitive processes, as well as regulation of cardiovascular control via changes to sympatho-vagal tone, and can be feasibly assessed using HRV in conjunction with more standardised behavioural outcomes. A frequency-domain HRV measure in the HF band, a marker of parasympathetic activity, was able to detect increased vagal activity both during, and in the 15 minutes following tDCS. Additionally, power in the LF band was found to correlate with changes in cognitive functioning, indicating an association between PFC activity and the sympathetic branch of the autonomic nervous system.”(1)
Jason Moore from Elite HRV describes:
Age
Age is one of the strongest factors that influences heart rate variability values. Lower heart rate variability generally indicates an increased biological age (older). Higher heart rate variability is correlated with increased fitness, health, and youthfulness.
It is well established that HRV is a measure of biological age. Biological age correlates heavily with homeostatic capacity, which is the body’s ability to self-stabilize in response to stressors. Studies have shown that biological age is a better measure for determining health status and risk than chronological age.
Chronological age correlates very strongly with biological age. However, there are now many communities that are discovering that lifestyle choices can strongly influence your biological age regardless of chronological age.
Since biological age is not easily quantifiable except via HRV measurement itself, here are some normal ranges for HRV based on chronological age according to the study analyzing 260 healthy subjects performed by Umetani et. al, 1998.
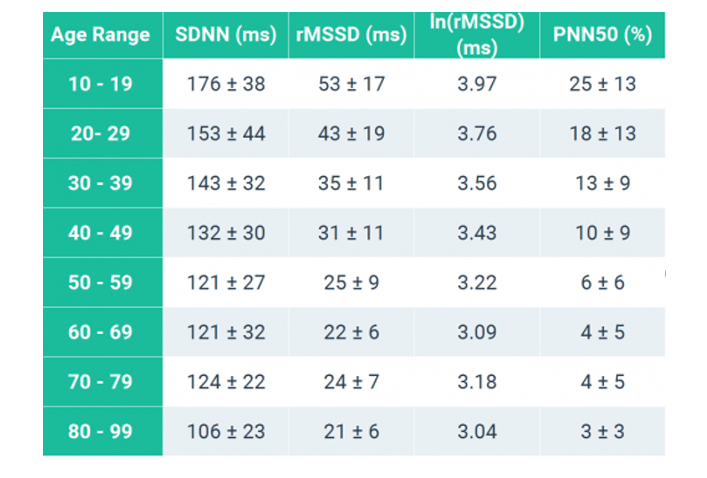
Gender
Although age and other factors play a stronger role in influencing HRV than gender, notable gender dependencies of short-term HRV indices have been observed.
The study “Short-Term Heart Rate Variability—Influence of Gender and Age in Healthy Subjects,” by Voss A et al. (2015) shows that males typically have lower Heart Rate Variability than females within the same age ranges. This indicates that males exhibit stronger Sympathetic (fight-or-flight stress response) tendencies over Parasympathetic (rest-and-digest) than comparable females.
In the study, the short-term (5 minute ECG/EKG measurements) HRV indices for 1,906 healthy individuals aged 25 – 74 years were analyzed to determine gender and age effects on HRV. The results showed that women under age 55 exhibit higher HRV time-domain values for RMSSD, PNN50, and, higher HF and lower LF and LF/HF ratio than men in comparable age ranges. In particular, the notably higher Time Domain RMSSD, lower Frequency Domain LF/HF ratio, and lower Poincare SD1/SD2 in females below the age of 55 demonstrate the increased Parasympathetic dominance.

Age and Gender
Interestingly, the gender-related variation in HRV decreases over the age of 55 years. The major age-related HRV differences for both genders are between ranges 35–44 years and 45–54 years. The study suggests that the gender-related HRV differences in the younger ages is probably caused by “the different hormonal situations leading to a higher sympathetic activity and a lower parasympathetic tone in men and vice versus in women”.
Furthermore, when comparing age groups 45–54 years and 55–64 years, females have significantly higher HRV differences compared to males, which corresponds to age-related hormone effects (menopause). The gender-related HRV differences disappear with aging because of the “hormonal restructuring” such as menopause in women and other hormonal changes in men that occur with aging and lead to more “comparable hormonal stages” in the gender groups above age 55.
Some other studies show that declined oestrogen levels associated with the Autonomic Nervous System changes seen in postmenopausal women (Moodithaya et al., 2009) and increased total HRV and baroreflex sensitivity in postmenopausal women with hormone replacement therapy (Pikkujämsä et al., 2001) suggest that hormonal factors play a role for the observed age and gender related differences.
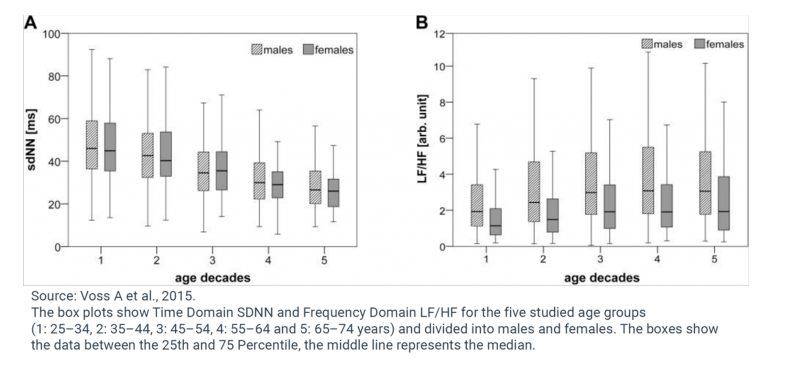
As can be seen from the data and discussed earlier, age strongly influences HRV, regardless of gender. Time Domain HRV indices decline and Frequency Domain LF and HF decrease while the LF/HF ratio increases with increasing chronological age for both genders (Agelink MW et al., 2001, Yukishita T et al., 2010, Greiser KH et al., 2009, and Felber Dietrich D et al., 2006). This age-dependent and gender-independent change to HRV indicates diminished Parasympathetic activation with increasing age.
Considerable loss of heart beat variability and complexity could mainly be caused by cardiovascular structural changes through the loss of sinoatrial pacemaker cells or decreased arterial flexibility and functional changes in other regulatory processes that occurs during aging (Ferrari AU et al., 2002).
So, while gender does influence HRV values, especially in younger years, age has a greater contribution to HRV and overall health and resilience.
Signals sent by the autonomic nervous system play tug of war with your heartbeat, speeding it up and slowing it down. The result is that your heart never beats evenly, varying its rhythm in response to everything.
The autonomic nervous system has two parts responsible for controlling your heartbeat: the sympathetic nervous system (SNS) and the parasympathetic nervous system (PNS) .
The PNS is the heart’s brake pedal
Your heart has a sinoatrial node (SA) and an atrioventricular node (AV).
The Vagus nerve, one of the central nerves of the parasympathetic nervous system, runs through the SA and AV nodes, like this.
When the PNS is activated, the impulses are generated less frequently and transmitting the signal from the SA to the AV takes longer
When the PNS is active, the heart beats less frequently and each beat lasts longer.
The SNS is the heart’s gas pedal
The walls of the heart are made of muscle cells, or cardiac myocytes.
These cells contract. When they contract, the heart contracts.
The nerves shown below belong to the sympathetic nervous system (SNS). They connected to cardiac muscle cells.
What RR intervals look like
When the SNS is activated, it boosts the production of ATP (an energy source) in the cardiac muscle cells, which means that the heart beats stronger. The cells in the heart activate, which means the impulses generated more quickly.
The SNS & PNS work together to help your body adapt to external changes in the environment. For example, when you work out, your muscles need more oxygen to perform. The SNS sends signals to increase the heart rate to deliver more blood to the muscles. When the workout is over, the PNS slows the heart rate down.
Brain control
This is when the brain’s subcortical structures are impacting heart function. They stimulate the hypothalamus and trigger reflexes, both of which activate the sympathetic nervous system. Parasympathetic nervous system activity is suppressed via reflex regulation and lower structures of the brain stem.
In short: The relaxing effects of the parasympathetic nervous system are suppressed and the sympathetic nervous system stimulates the heart. This stimulation comes from two key structures — the subcortical structures and the hypothalamus.
Moderate brain control
This is when the hypothalamus is primarily responsible for heart regulation. It triggers reflexes that activate the sympathetic nervous system. Depending on the situation, these reflexes can activate the parasympathetic nervous system as well.
So the sympathetic nervous system is constantly active, and is periodically balanced out by the parasympathetic nervous system.
In short: The heart receives an equal number of stimulating and relaxing signals.
Auto control
The heart operates autonomously via impulses sent by the sinoatrial node (SA) and its activity is only modified by the parasympathetic nervous system.”(2)
References:
1. Nikolin S, Boonstra TW, Loo CK, Martin D. Combined effect of prefrontal transcranial direct current stimulation and a working memory task on heart rate variability. PLoS One. 2017 Aug 3;12(8):e0181833. doi: 10.1371/journal.pone.0181833. PMID: 28771509; PMCID: PMC5542548.
2. Jason Moore, HRV Demographics 2016. https://hrvcourse.com/hrv-demographics-age-gender/?afmc=go
3. Umetani K, Singer DH, McCraty R, Atkinson M. Twenty-four hour time domain heart rate variability and heart rate: relations to age and gender over nine decades. J Am Coll Cardiol. 1998 Mar 1;31(3):593-601. doi: 10.1016/s0735-1097(97)00554-8. PMID: 9502641.
4. Voss A, Schroeder R, Heitmann A, Peters A, Perz S. Short-term heart rate variability--influence of gender and age in healthy subjects. PLoS One. 2015 Mar 30;10(3):e0118308. doi: 10.1371/journal.pone.0118308. PMID: 25822720; PMCID: PMC4378923.
Comments